Table of Contents
Introduction: A Growing Need for Waste-to-Energy (WTE)
Picture a world struggling under the weight of overflowing landfills, polluted water sources, and an ever-growing demand for cleaner energy solutions. This grim reality underscores why Waste-to-Energy (WTE) Technologies & Applications have become a beacon of hope for governments, industries, and communities around the globe. Within the first hundred words of this guide, If you’re curious about how garbage can be converted into electricity or how trash might soon power entire neighborhoods, keep reading. This in-depth exploration will arm you with the knowledge, strategies, and real-world examples you need to understand and perhaps champion WTE for a more sustainable future.
In this article, you’ll discover:
- The global waste crisis and how WTE offers viable solutions
- Different WTE technologies, from incineration to advanced plasma arc gasification
- Real-world case studies such as the Lostock Incinerator, Auburn Waste to Energy, and Greenspot Waste to Energy projects
- The process of setting up a WTE Plant and the obstacles faced
- Environmental, economic, and societal impacts of turning trash into electricity
- Best practices, expert insights, and actionable steps to leverage WTE infrastructures
By the end, you’ll grasp not only why waste-to-energy is crucial for modern civilization but also how you can support or implement it in your community or business. Ready to turn trash into treasure? Let’s dive in.
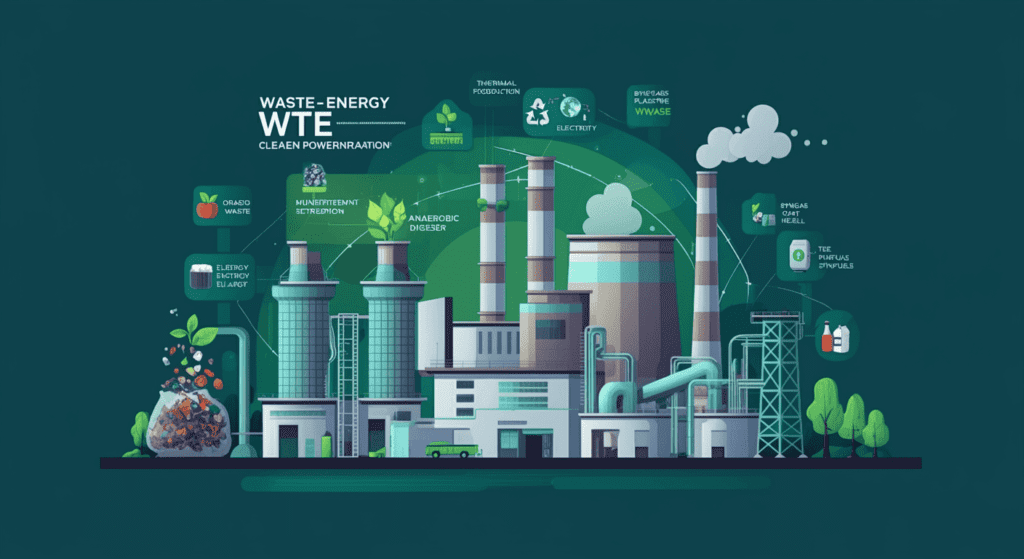
Understanding the Global Waste Challenge
To appreciate the significance of Waste to Energy (WTE), one must first understand the magnitude of the waste problem. Mountains of garbage continue to grow worldwide, with population spikes and consumer culture accelerating the pace. This section breaks down the depth of our collective trash dilemma and why it calls for robust solutions like WTE.
The Scale of the Problem
According to the World Bank, global annual waste generation could surpass 3.4 billion tons by 2050, a stark rise from the current estimates of about 2 billion tons. Traditional approaches to waste management—landfills and open dumping—are no longer sufficient, nor are they environmentally sound. In many countries, limited space for landfills and escalating environmental regulations have forced authorities to seek next-generation solutions such as Waste to Energy.
Landfill Overload
Landfills take up vast amounts of land and, when poorly managed, can contaminate air, soil, and water. Traditional dumps also emit significant volumes of methane—a greenhouse gas more potent than carbon dioxide. While landfill gas recovery systems can capture some of these emissions and even convert them to energy, the efficiency is often too low to solve the broader waste problem.
Environmental Impact
The ecological fallout from trash accumulation includes:
- Soil Degradation: Toxic substances can leach into the soil, hampering agriculture and biodiversity.
- Water Contamination: Leachate—a liquid formed when rainwater filters through waste—can pollute nearby rivers, lakes, and groundwater.
- Air Pollution: Landfills often release harmful gases such as methane and volatile organic compounds (VOCs), which can degrade air quality and harm public health.
These mounting environmental challenges have made Energy from Waste a compelling proposition: instead of letting trash decompose and pollute the environment, we can harness its energy potential.
What Is Waste-to-Energy (WTE)?
Waste-to-Energy (WTE), also referred to as Energy and Waste or Trash to Energy, is an umbrella term for technologies that convert solid waste into usable forms of energy—electricity, heat, or fuel. At its core, WTE addresses two global imperatives: mitigating the environmental issues arising from landfills and providing an alternative to fossil fuels.
Definition & Core Principles
The principle behind WTE Energy is surprisingly straightforward. Waste comprises organic materials—paper, food scraps, wood, textiles, and even some plastics—that still hold latent energy. Rather than letting garbage rot in landfills, WTE plants subject it to processes that break down these organic molecules, releasing energy in a controlled manner. This energy can then be harnessed as electricity or heat, or captured in fuels like biogas or syngas.
Why WTE Matters for Sustainability
- Resource Efficiency: WTE promotes a circular economy by extracting as much value as possible from waste before final disposal of any remaining ash or non-burnable residues.
- Emissions Reduction: Modern WTE plants use advanced pollution control technologies—such as flue-gas treatment and high-temperature combustion—to significantly reduce harmful emissions, thereby outperforming traditional incineration methods of the past.
- Energy Security: As countries seek energy independence, WTE offers a local, continuous source of power, mitigating reliance on imports of oil, coal, or natural gas.
Beyond these tangible benefits, a well-implemented Waste to Electricity Plant can become a catalyst for sustainable development, reducing environmental burdens and driving innovation in technology, policy, and education.
WTE Technologies: Core Mechanisms of Energy Recovery
Waste-to-Energy isn’t a single process but a diverse range of technologies designed to harness energy from various types of waste. Below are the most prevalent methods and how they work. Understanding these processes can help stakeholders decide which technology best suits their WTE Infra needs.
Incineration: The Traditional Backbone
Incineration is the most widely recognized form of WTE. It involves combusting waste at high temperatures—around 850°C (1,562°F) or higher—in specialized furnaces. The heat generated produces steam, which drives turbines to generate electricity.
- Advantages:
- Reduces waste volume by up to 90%
- Straightforward and proven technology
- Can handle mixed municipal waste streams
- Drawbacks:
- Requires robust pollution control to manage emissions
- Ash disposal remains an issue, as it can contain heavy metals and other toxins
- Public perception challenges, especially regarding air quality
Despite these challenges, modern incineration plants—such as the Lostock Incinerator in the UK—have implemented sophisticated filters, scrubbers, and electrostatic precipitators to minimize harmful emissions. The result is a cleaner, high-volume solution for communities seeking to reduce landfill reliance.
Gasification: Converting Solid Waste to Syngas
Gasification breaks down organic matter at high temperatures in a low-oxygen environment to produce syngas, a mixture of carbon monoxide (CO) and hydrogen (H₂). This syngas can then be burned to produce electricity or further refined into chemicals or fuels.
- Advantages:
- Produces a cleaner fuel (syngas) for flexible uses
- Generates fewer pollutants than traditional incineration
- Higher energy efficiency potential
- Drawbacks:
- Requires uniform waste feedstock for optimal efficiency
- Technology can be costly and complex
- Operational expertise and rigorous maintenance are essential
Gasification exemplifies the push toward advanced, multi-step waste conversion processes and is gaining attention for its potential to integrate seamlessly with hydrogen economy initiatives.
Pyrolysis: Harnessing Heat in the Absence of Oxygen
Pyrolysis involves heating waste in an oxygen-free environment. This process breaks down waste into various byproducts:
- Biochar: A carbon-rich solid
- Pyrolysis Oil: A liquid fuel that can be refined
- Synthetic Gas: A gaseous byproduct that can be used for heating or electricity generation
Pyrolysis is often lauded for its potential to produce valuable biochar, which can improve soil fertility and sequester carbon. However, like gasification, it demands consistent waste streams and high operational costs.
Anaerobic Digestion: Biogas from Organic Waste
Anaerobic digestion (AD) targets biodegradable waste, such as food scraps, agricultural residues, and wastewater sludge (often referred to as WTE Water in some contexts). In an oxygen-deprived environment, microorganisms break down organic matter to produce biogas—a mix of methane (CH₄) and carbon dioxide (CO₂).
- Advantages:
- Ideal for wet, organic-rich waste streams
- Produces digestate, a nutrient-rich residue that can be used as fertilizer
- Lower emissions and odors compared to open composting
- Drawbacks:
- Not suitable for non-organic or heavily contaminated waste
- Requires consistent temperature and moisture levels for bacteria to thrive
- Slower process compared to thermal methods
In many cities, Anaerobic Digestion is a cornerstone of integrated waste management, especially when dealing with municipal wastewater or agricultural byproducts.
Plasma Arc Gasification: Cutting-Edge but Costly
A more advanced variant of gasification, plasma arc gasification employs an electrical arc to generate plasma at temperatures exceeding 4,000°C (7,232°F). This extreme heat disintegrates waste into its atomic components, producing syngas and a glass-like slag that can be used in construction.
- Advantages:
- Capable of destroying hazardous and medical waste
- Minimal emissions with proper gas clean-up
- Produces an inert slag that can be repurposed
- Drawbacks:
- Extremely high energy input, leading to high operational costs
- Complex technology requiring skilled personnel
- Still evolving and less proven at large commercial scales
Despite the hurdles, plasma arc gasification represents a frontier in WTE Technologies. It often appeals to research institutions and specialized facilities seeking near-complete waste destruction and minimal byproducts.
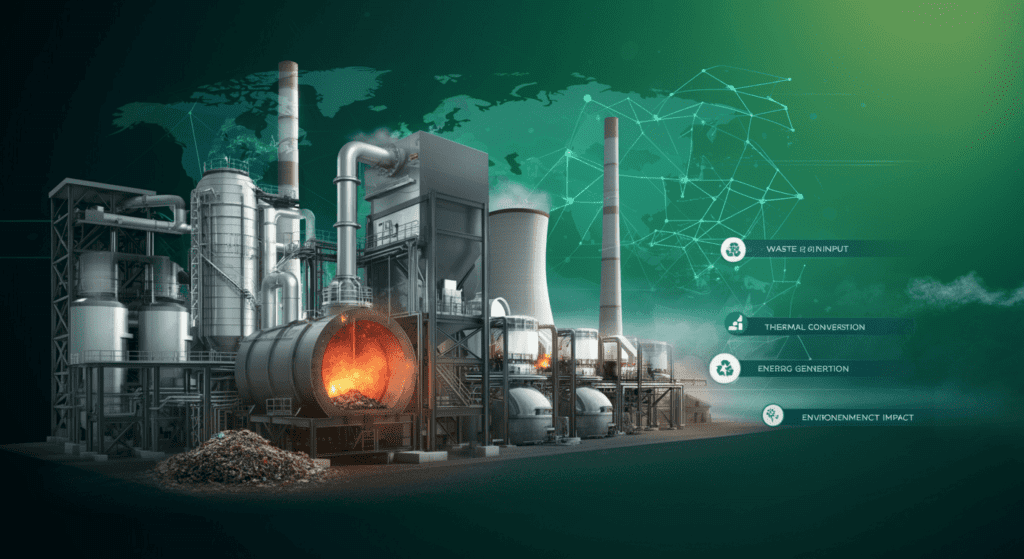
Real-World WTE Applications and Case Studies
Concrete examples underscore the success stories behind Waste to Energy Plants. From large-scale incinerators powering entire cities to smaller community-based digesters, WTE projects worldwide showcase diverse outcomes and lessons learned.
Lostock Incinerator in the United Kingdom
The Lostock Incinerator in Northwich, UK, stands as a prime example of a modern, large-scale WTE facility. Equipped with advanced flue-gas cleaning systems, it aims to minimize dioxins and other pollutants. The plant’s dual-line combustion system can process over 600,000 tons of residual waste annually, producing enough electricity to power tens of thousands of homes.
- Notable Insights:
- Community engagement was crucial: public consultations and transparent emission reporting helped build local trust.
- Emphasis on ash management: leftover bottom ash finds use in road construction, significantly reducing the volume heading to landfill.
Auburn Waste to Energy in the United States
The Auburn Waste to Energy project in Maine (often cited for its combination of incineration and resource recovery) has focused heavily on sustainable ash disposal and metal recovery. By sorting metals before combustion, the facility recovers valuable materials like aluminum and steel, selling them for recycling. After combustion, non-ferrous metals are extracted from the bottom ash, further boosting resource efficiency.
- Notable Insights:
- Integration with local recycling programs ensures that only non-recyclable waste feeds the incinerator.
- Continuous emission monitoring fosters transparency and adherence to stringent U.S. EPA standards.
Greenspot Waste to Energy in the Netherlands
The Greenspot Waste to Energy initiative represents a synergy between anaerobic digestion and composting. Targeting organic waste from households, restaurants, and farms, the facility transforms food scraps into biogas and nutrient-rich digestate. The generated biogas powers nearby industrial processes and supplies heat to greenhouses, aligning with the Netherlands’ push toward a circular economy.
- Notable Insights:
- Co-location of greenhouses takes advantage of surplus heat, elevating overall system efficiency.
- Public acceptance soared due to the project’s explicit focus on reducing odors, greenhouse gas emissions, and reliance on chemical fertilizers.
Each of these examples highlights that Waste-to-Energy is not a one-size-fits-all approach. Instead, local factors—including waste composition, regulatory framework, and community sentiment—shape a WTE project’s design and performance.
WTE Infrastructure & Implementation Challenges
Establishing a Waste to Electricity Plant or integrated WTE program involves more than just technical design. Policy, financing, social acceptance, and operational details can either propel or sink a project. Below is a closer look at these pivotal factors.
Key Steps in Building a WTE Plant
- Feasibility Study
- Assess waste composition and volumes.
- Evaluate technology suitability (incineration, gasification, or anaerobic digestion).
- Forecast economic returns and environmental impacts.
- Permits & Regulations
- Comply with local, regional, and national environmental laws.
- Secure necessary air, water, and land use permits.
- Demonstrate compliance with emissions standards, often measured in micrograms per cubic meter for specific pollutants.
- Design & Engineering
- Collaborate with specialized engineers for custom systems.
- Address site-specific concerns, such as potential odor or noise.
- Integrate robust safety measures for plant workers.
- Construction & Commissioning
- Erect the main plant structure, install technology modules (boilers, turbines, digesters, etc.).
- Conduct test runs to ensure everything functions correctly.
- Operations & Maintenance
- Employ trained technicians to oversee day-to-day processes.
- Regularly monitor emissions and equipment performance.
- Establish contingency plans for potential downtime.
Policy & Regulatory Hurdles
A robust regulatory framework can either accelerate or impede WTE implementation. Some regions offer tax incentives or renewable energy credits for electricity produced from waste, recognizing its alignment with climate goals. Conversely, certain jurisdictions face intense environmental lobby pressure, leading to stricter emission limits or moratoriums on new incinerators.
- Policy Trends:
- Mandated waste sorting at the source to improve feedstock quality.
- Cap-and-trade programs that penalize high carbon emitters, effectively advantaging lower-emission WTE solutions.
- Extended producer responsibility (EPR) laws that encourage manufacturers to reduce packaging waste.
Cost & Financing
Building a WTE Plant can be capital-intensive, costing anywhere from a few million to over a billion dollars for large-scale projects. Financing often involves a mix of public funds, private investments, and development grants. Investors seek long-term contracts—known as Power Purchase Agreements (PPAs)—to ensure stable returns.
- Financial Instruments:
- Green bonds, attracting environmentally focused investors.
- Public-private partnerships (PPPs), distributing risk and responsibility.
- Multilateral development bank loans, especially in emerging markets aiming to modernize waste management.
Public Perception & Community Engagement
No technology can thrive without community buy-in. Concerns about air pollution, odor, and health risks can derail the best-planned projects if not properly addressed. Successful WTE ventures often prioritize:
- Transparent Communication: Regular updates, open-door policies, and data sharing on emissions.
- Community Benefits: Offering discounted electricity rates, job creation, or improved local infrastructure.
- Inclusive Planning: Involving local stakeholders—residents, NGOs, businesses—early in the decision-making process.
Environmental Impact & Sustainability Considerations
Although Waste to Energy can substantially reduce landfill dependency and cut greenhouse gas emissions, it’s not without its controversies. Critics argue that incineration may undermine recycling efforts, while proponents highlight WTE’s minimal land footprint compared to landfilling.
Emission Controls: Ensuring Clean Air
Modern WTE facilities employ multi-stage emission control systems:
- Electrostatic Precipitators capture fine particulates.
- Scrubbers remove acidic gases (e.g., sulfur dioxide).
- Activated Carbon Injection absorbs mercury, dioxins, and furans.
- Selective Catalytic Reduction (SCR) curbs nitrogen oxides (NOₓ).
When designed and managed effectively, these technologies reduce pollutants to levels comparable to—or sometimes lower than—coal-fired power plants of the same capacity.
Recycling vs. Incineration: Finding the Right Balance
Critics often claim WTE discourages recycling, fearing communities may opt to burn recyclables for energy. However, leading WTE projects prioritize a waste hierarchy:
- Reduce consumption
- Reuse materials
- Recycle whenever possible
- Recover Energy through WTE
- Dispose only what remains
This hierarchy places WTE as a last resort for non-recyclable waste, ensuring that incineration does not cannibalize recycling efforts but complements them by handling residual waste.
Best Practices for Sustainability
- High-Quality Feedstock: Sort waste at source to remove recyclables, hazardous materials, and high-value organics for separate processing.
- Continuous Monitoring: Deploy digital sensors and real-time data analytics for emissions and operational parameters.
- Ash Utilization: Explore beneficial reuse of bottom ash in construction or road-building to minimize landfilling.
- Stakeholder Collaboration: Build cross-sector partnerships to unify regulation, finance, and technology development.
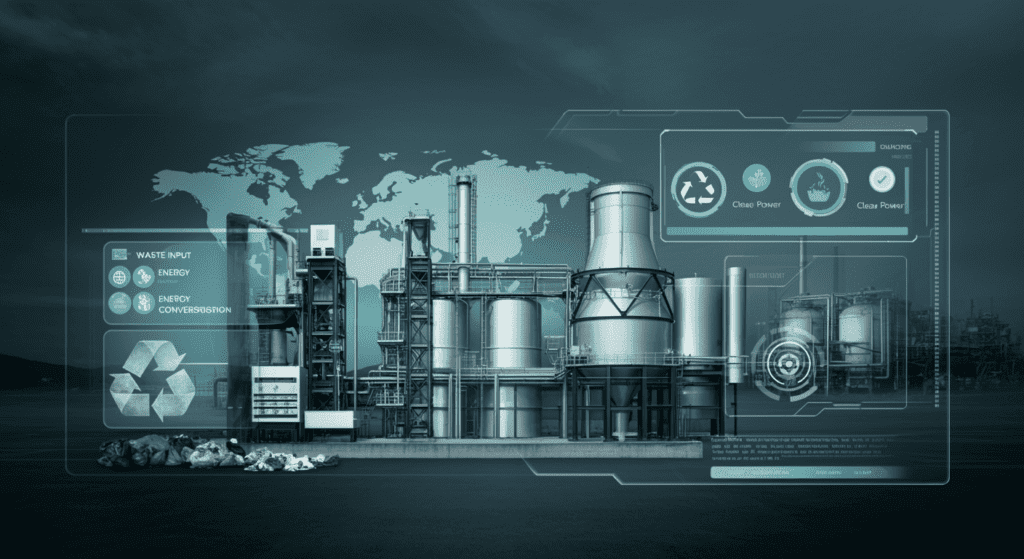
Future Trends in WTE
The Waste-to-Energy (WTE) sector is evolving, driven by technological advancements, policy changes, and shifting global perspectives on sustainability. Below are some trends shaping the future of trash-to-power initiatives.
Technological Innovations
- Advanced Gas Cleaning: New sorbent materials and catalysts aim to capture even trace pollutants at higher efficiencies.
- AI-Driven Facility Management: Machine learning algorithms can optimize combustion parameters and maintenance schedules, boosting plant efficiency and cutting costs.
- Hybrid Systems: Facilities that combine multiple WTE methods (e.g., gasification + anaerobic digestion) for greater flexibility and resource recovery.
Integration with Circular Economy
As governments and industries pivot toward a circular economy, WTE finds its place alongside reuse, recycling, and composting. For instance, a community could first remove all recyclables and then channel biodegradable waste to anaerobic digestion facilities while sending only residual non-recyclable items to an incinerator or gasifier.
WTE in Developing Regions
Rapidly urbanizing areas in Asia, Africa, and Latin America are adopting WTE to address a lack of landfill space and to manage mounting waste volumes. However, these projects often grapple with issues such as inconsistent waste composition, limited technical expertise, and financial hurdles. Partnerships with international organizations, plus capacity-building for local engineers and technicians, can help ensure longevity and success.
Frequently Asked Questions (FAQ)
Q1: Is WTE truly eco-friendly, or does it produce harmful emissions?
Modern Waste-to-Energy facilities incorporate state-of-the-art emission control technologies—scrubbers, filters, and catalytic converters—to substantially minimize pollutants. When regulated properly, WTE plants can maintain emissions within strict limits and often outperform older coal- or oil-fired power plants in terms of air quality impact.
Q2: What’s the difference between a WTE plant and a traditional incinerator?
A traditional incinerator primarily aims to reduce waste volume without generating substantial energy. A WTE Plant not only combusts waste but also captures and converts the released heat into electricity or steam. Additionally, WTE facilities feature sophisticated pollution control systems that differentiate them from older, simpler incinerators.
Q3: Are recycling rates affected by WTE facilities?
In well-structured waste management systems, WTE is positioned below recycling and composting in the waste hierarchy, handling only the residual waste. This approach preserves high recycling rates by prioritizing material recovery before energy recovery.
Q4: How expensive is it to build a WTE facility?
Costs vary widely based on technology type, capacity, and regional regulations. Capital investments can range from tens of millions for smaller anaerobic digestion facilities to over a billion dollars for large-scale incineration or plasma gasification plants. Financing often involves public-private partnerships, government subsidies, and long-term power purchase agreements.
Q5: What role can communities play in supporting WTE?
Communities can advocate for responsible waste management, segregate their waste at source, and participate in public consultations. Local buy-in is crucial for gaining the social license to operate. Moreover, communities can help track and report any operational concerns, ensuring transparency and continuous improvement.
Conclusion: Shaping a Cleaner Future with Waste-to-Energy
The global quest to manage the ever-increasing mountain of garbage—while also securing reliable, cleaner energy—places Waste-to-Energy (WTE) Technologies & Applications at the forefront of sustainable solutions. Whether it’s the incineration-based Lostock Incinerator in the UK, the Auburn Waste to Energy facility in the United States, or the Greenspot Waste to Energy project in Europe, each offers a practical blueprint for transforming what many see as worthless “trash” into a stable, renewable resource. By aligning advanced technologies with stringent emission controls, robust financial backing, and strong community engagement, WTE holds the promise of cutting landfill volumes, reducing carbon footprints, and contributing to energy security worldwide.
As you’ve learned throughout this extensive guide, Waste-to-Energy (WTE) Technologies & Applications epitomize the next phase of sustainable waste management and energy generation. By leveraging incineration, gasification, pyrolysis, or anaerobic digestion, we can divert monumental amounts of trash from landfills while generating electricity, heat, or valuable fuels—effectively turning a vexing environmental challenge into an opportunity for progress.
If you’re ready to champion these methods or explore them for your community or organization, the time to act is now. Share this article, post your questions, and spread the word about how trash can indeed become treasure. Together, we can turn the tide on waste and steer the planet toward a cleaner, more resilient future.
Ready to Dive Deeper or Take Action?
- Comment below with your thoughts or any WTE projects in your area.
- Share this guide to raise awareness about turning waste into energy.
- Subscribe to our newsletter for updates on WTE innovations, policy shifts, and real-world success stories.
- Explore Related Resources on our site for more on waste management, recycling, and environmental policies.
Remember, every piece of trash you throw away could be a potential energy source. Let’s seize that opportunity today.
Stay Connected with EngiTech
EngiTech is your trusted source for in-depth knowledge on industrial mechanical engineering machines and technologies. Stay ahead with the latest innovations, expert insights, and practical guides designed to help you make informed decisions for your business and engineering needs. Join our growing community of professionals and industry leaders to stay updated and competitive in the ever-evolving world of industrial technology.